Today I had the opportunity to present UMPSA STEM Lab’s work on – Exploring the Impact of Arduino Robotics Instruction on Physical Computing and Programming Skills, at the 2024 Research and Education in Mechatronics (REM) Conference. This paper highlights the role of Arduino-based robotics education in enhancing engineering students’ programming, physical computing, and problem-solving abilities.
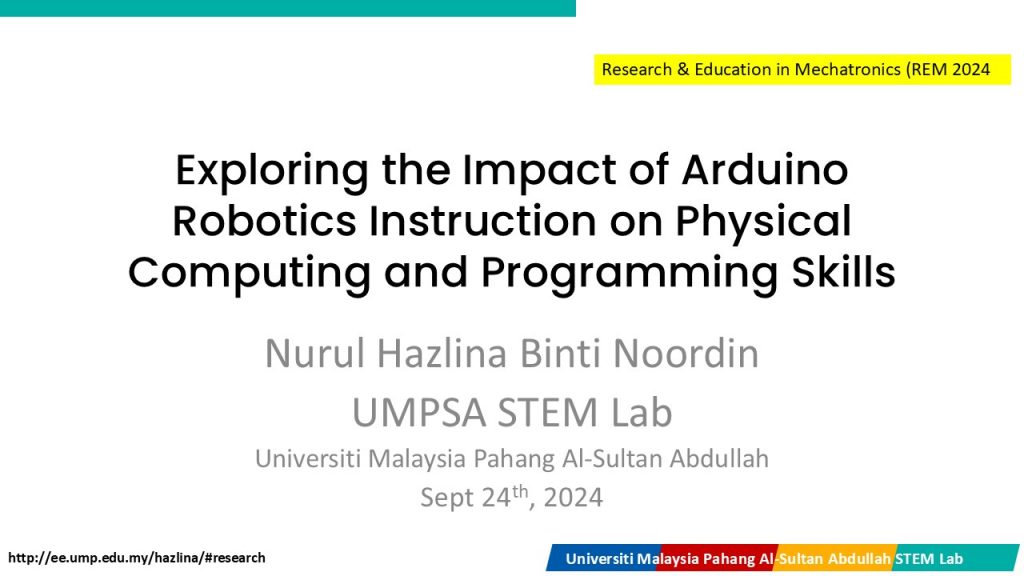
In the study, we found significant improvements in students’ performance after participating in the Arduino Robotics Module.
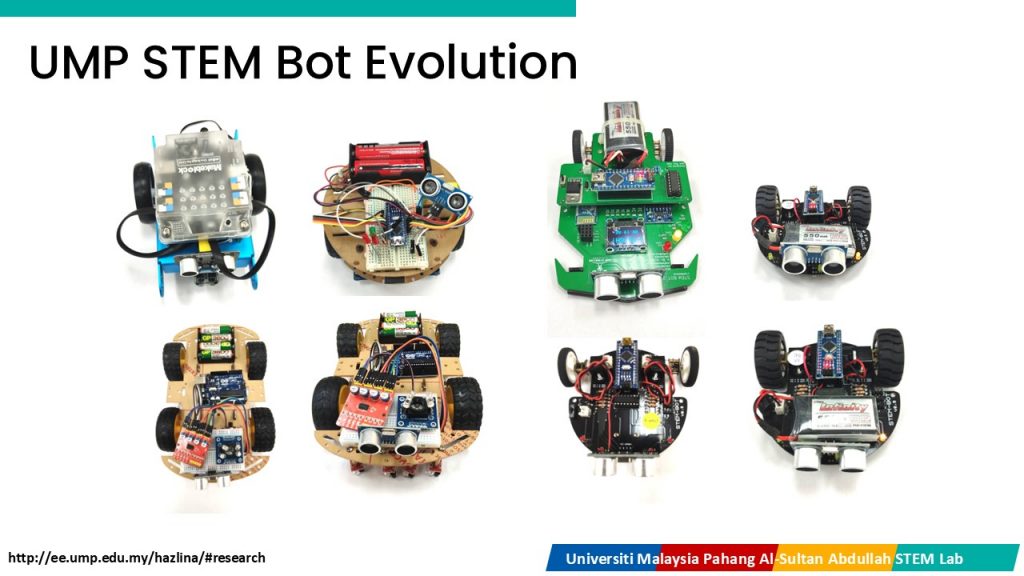
By comparing pre-test and post-test assessments, students demonstrated an average improvement of 23.24 percentage points in areas like coding proficiency, electronics, and robotics. This shift highlights how hands-on, project-based learning enhances not only theoretical knowledge but also practical skills, which are crucial in engineering education.
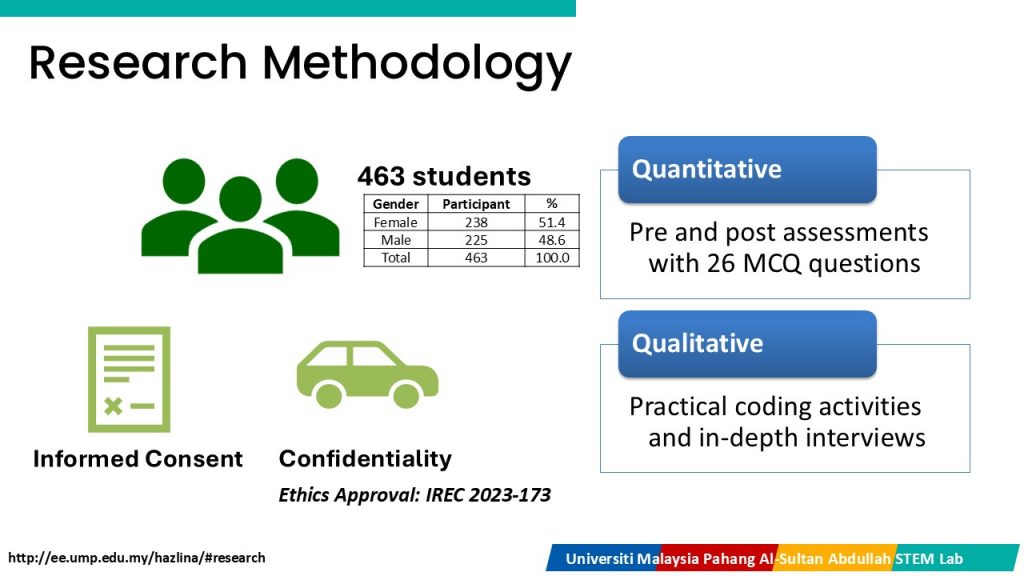
The results were further validated through paired T-test analysis, which showed statistically significant improvements (p-value < 0.001), confirming that the Arduino module had a substantial impact on students’ learning outcomes. This analysis is important in educational research settings as it provides a rigorous method of assessing whether the changes observed are truly due to the educational intervention and not just random variation.
The study employed a mixed-methods approach, combining both quantitative (pre-test/post-test assessments) and qualitative (focus group discussions, surveys) data. In educational research setting, using mixed methods is essential as it allows for a more comprehensive understanding of the students’ learning experiences.
- Quantitative Data – The pre- and post-tests provided measurable insights into the knowledge gained by students. The use of a paired T-test helped in statistically validating the learning improvements.
- Qualitative Data – Focus group discussions offered deeper insights into students’ perceptions and challenges faced during the module. This data helped contextualize the numbers by revealing students’ experiences, confidence levels, and the areas they found most beneficial or difficult.
Using a mixed-methods approach is especially valuable in the context of engineering education. It combines the objectivity of numerical data with the richness of personal feedback, ensuring that both learning outcomes and student experiences are thoroughly evaluated. This method offers a holistic view of the educational intervention’s effectiveness, making it ideal for complex subjects like robotics education.
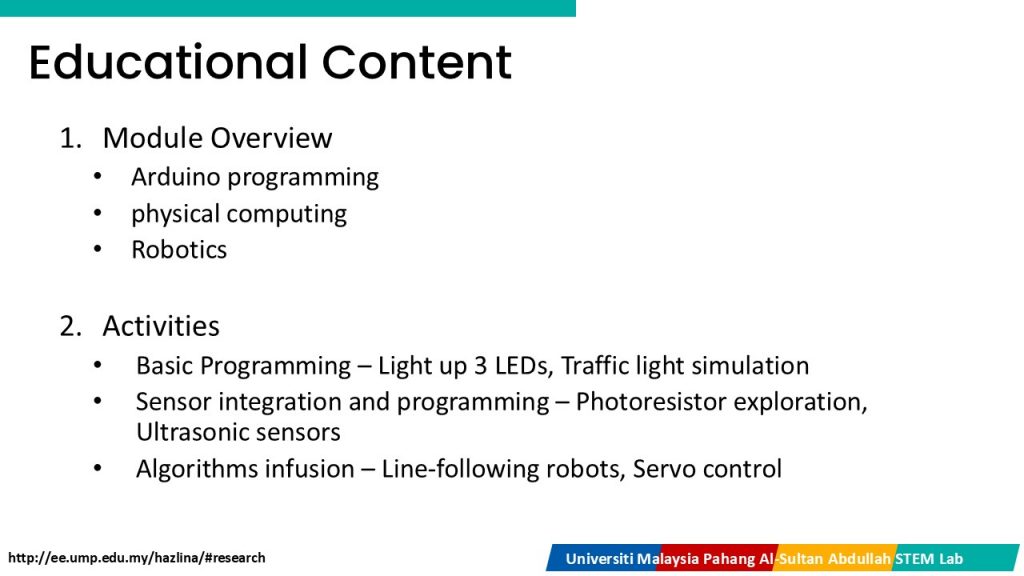
As part of this research, students engaged in a series of structured activities that spanned programming, electronics, and embedded systems with robotics. These activities were designed to develop essential skills in each domain:-
A. Programming – Students wrote and debugged code for various tasks using the Arduino platform.
Key programming activities included:
-
- Lighting up LEDs – Basic digital and analog I/O programming.
- Traffic light simulation – Students programmed an automated traffic light using conditional statements and timers.
- OLED display and sensor integration – Students coded Arduino to interact with sensors and display outputs on OLED screens.
Programming is foundational for engineering students, especially in mechatronics. Mastery of programming helps students understand the logic behind automation and control systems, making them capable of designing software solutions for embedded systems.
B. Electronics and Embedded Systems – Students explored physical computing by connecting and controlling electronic components such as photoresistors, ultrasonic sensors, and servos with Arduino.
Activities included:
- Photoresistor experiments – Students measured light intensity and converted it into electrical signals.
- Ultrasonic sensor integration – Students used sensors to detect object distance and trigger actions like LED or motor control.
Electronics form the backbone of physical computing. Understanding circuits and sensors empowers students to design systems that interact with the real world, crucial for embedded systems development. These skills are indispensable for engineers working with IoT and smart devices.
C. Robotics – In the final phase, students applied their programming and electronics knowledge to design and build autonomous robots.
Key activities included:
- Line-following robots -Students programmed robots to follow a path autonomously using sensors.
- Obstacle avoidance – Robots were equipped with ultrasonic sensors to detect and avoid obstacles during movement.
Robotics brings together various engineering disciplines—mechanical, electrical, and software—allowing students to see how theory translates into practice. It also enhances critical thinking and problem-solving skills, which are vital for engineering graduates facing real-world challenges.
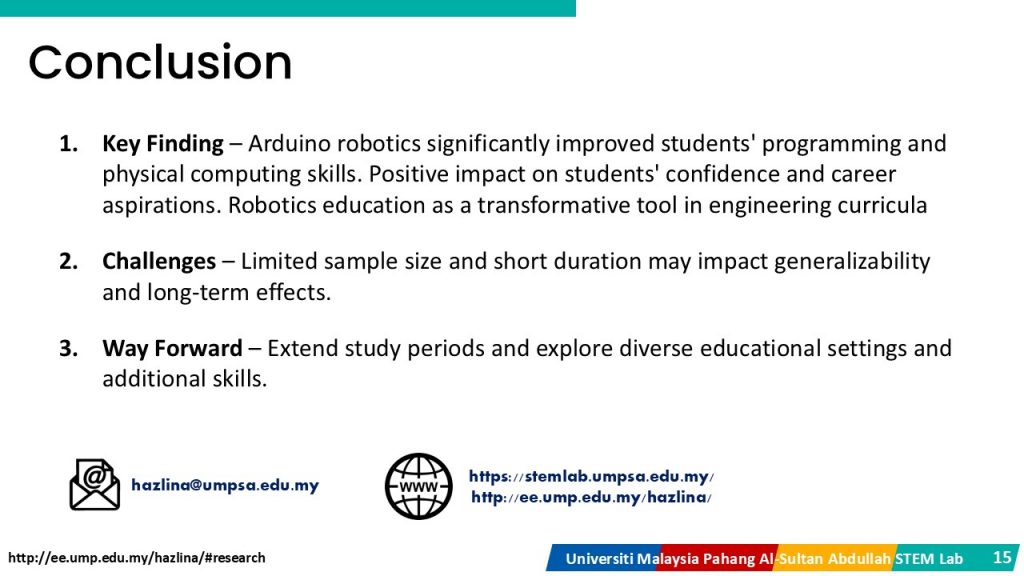
This marks the second article on engineering education from the UMPSA STEM Lab in 2024, continuing the mission to enhance engineering education. I strongly believe that being involved in research in engineering education is essential, as it helps to continuously improve teaching methodologies, ensuring that students are equipped with both theoretical and practical skills needed in today’s rapidly evolving technological landscape. With industries increasingly leaning on automation, robotics, and embedded systems, educational interventions like the Arduino Robotics Module bridge the gap between academia and industry requirements.
Educational research also allows educators to measure the effectiveness of new pedagogical approaches, providing insights into how students learn best. In this study, not only the improvements in technical skills are observed but also a marked increase in student confidence, engagement, and interest in the subject are seen.
Figure of Merit T-Test
In doing this research, I learned about the T-test. As discussed in the paper, the T-test revealed a mean improvement of -23.24 in students’ test scores, with a confidence interval confirming that the improvement was statistically significant and not due to random variation.
The T-test is a critical tool in educational research because it provides an objective way to measure the effectiveness of an intervention—in this case, the Arduino Robotics Module. By comparing pre- and post-test results, the T-test demonstrates with statistical certainty that the module genuinely enhanced students’ knowledge and skills. This method not only quantifies the improvement but also provides educators and researchers with concrete evidence of the program’s impact, enabling them to make informed decisions about refining or expanding similar educational initiatives in the future.
A T-test is a statistical method used to determine if there is a significant difference between two sets of data, like students’ scores before and after an educational intervention. To calculate a T-test, you first find the difference between each student’s pre-test and post-test scores. Then, you calculate the average of these differences and determine how much the differences vary (this is called the standard deviation). Using these values, the T-test formula calculates a t-value that shows how large the improvement is compared to random variation. The T-test also considers the sample size (number of students, in this case 463 students) to assess how reliable the result is.
Another important parameter in the T-test is the p-value, which indicates whether the improvement is statistically significant—meaning it likely didn’t happen by chance. In educational research, a low p-value (usually less than 0.05) means the intervention, such as a new teaching method or tool, genuinely improved students’ learning. This helps educators understand the real impact of their teaching strategies and make decisions about future programs.
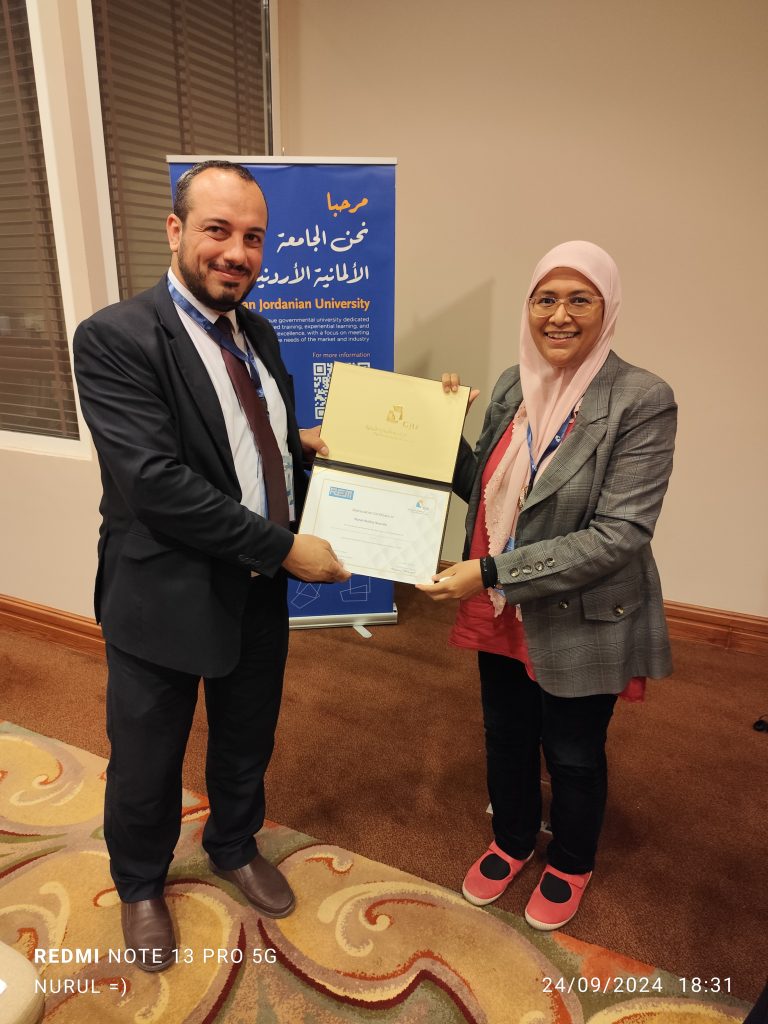
The findings from this study have far-reaching implications for the engineering education community. As technology advances, it’s becoming increasingly important to equip students with not just theoretical knowledge but also practical, hands-on experience. The Arduino Robotics Module bridges this gap by providing an interactive platform where students can apply engineering concepts in real-world contexts.
Integrating robotics and physical computing into the curriculum enhances student engagement, fosters creativity, and improves problem-solving skills. These attributes are essential in preparing future engineers to tackle the challenges of a rapidly evolving technological landscape.
I’m honored to present this work at the 2024 REM Conference and to contribute to the growing body of research in engineering education. I look forward incorporating more hands-on, interactive modules in my teaching, and to leverage the power of robotics in developing critical engineering skills, InSyaAllah
Illaliqa’ 🙂
Nurul – Jordan Sept 24th
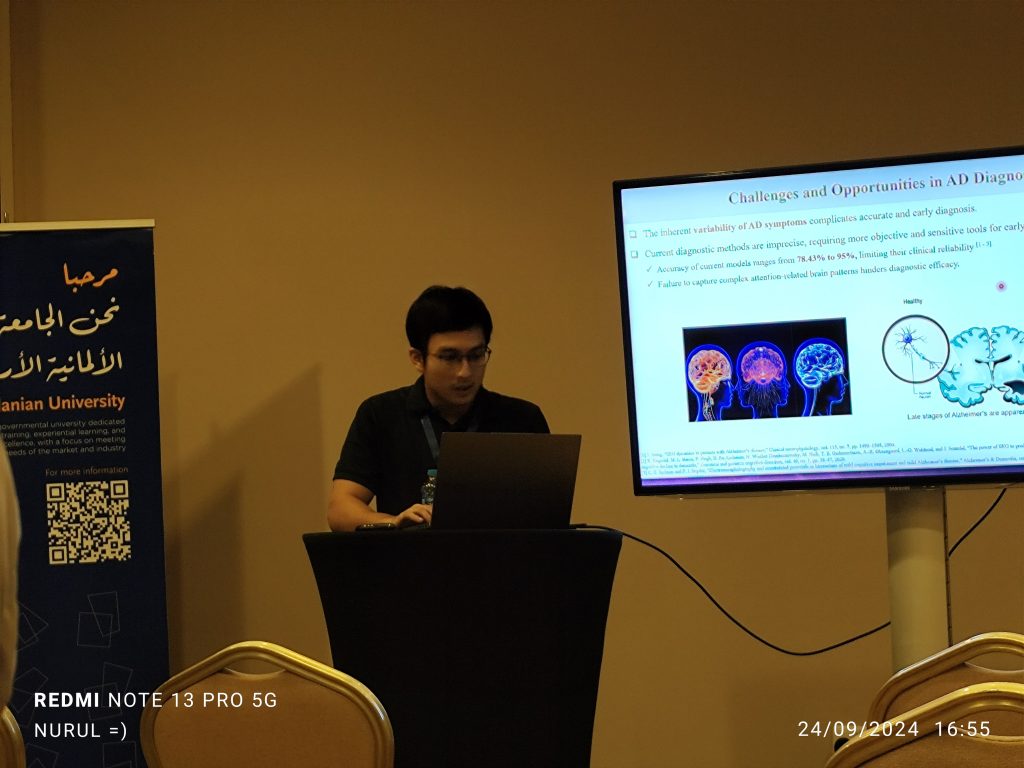
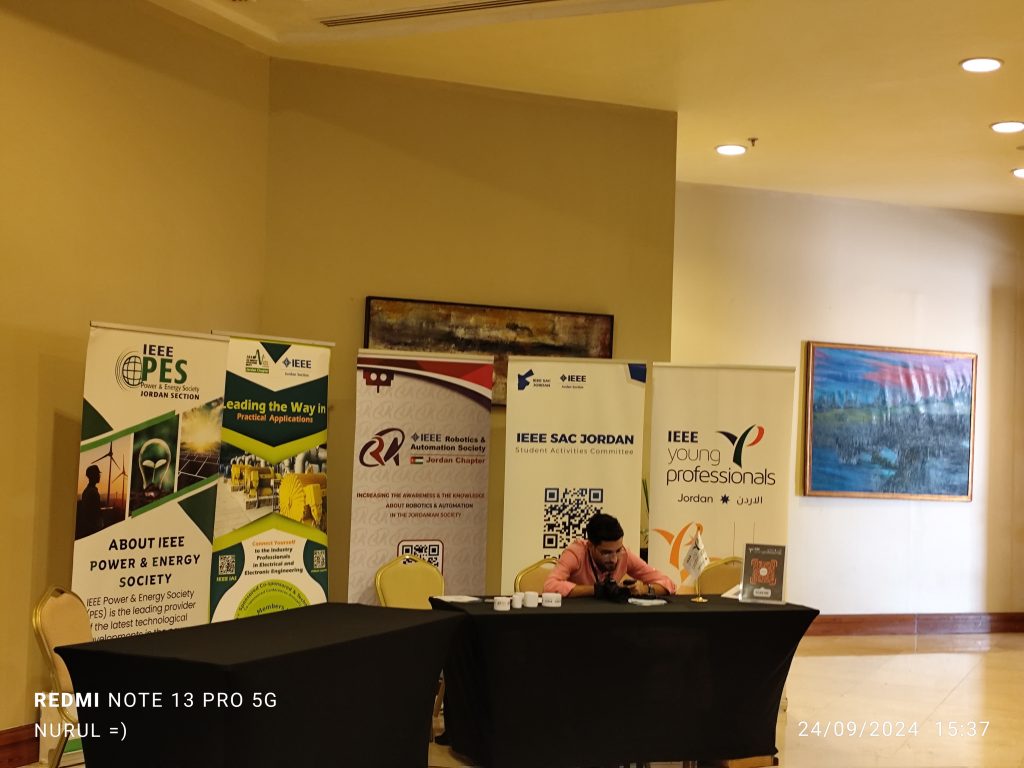
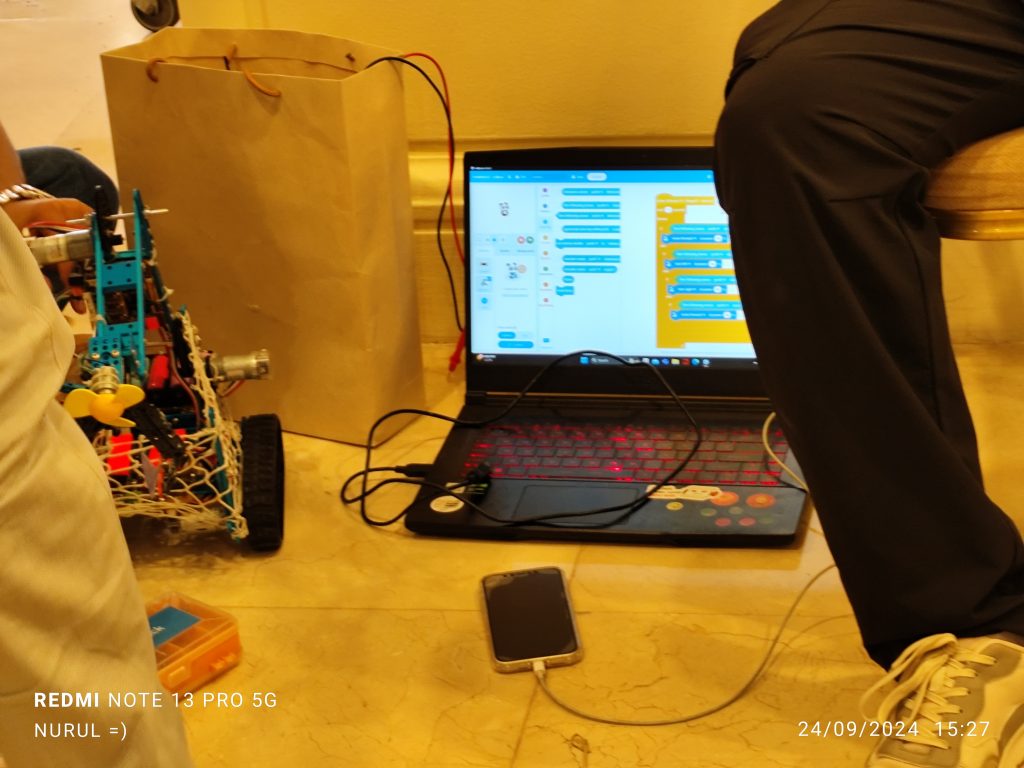
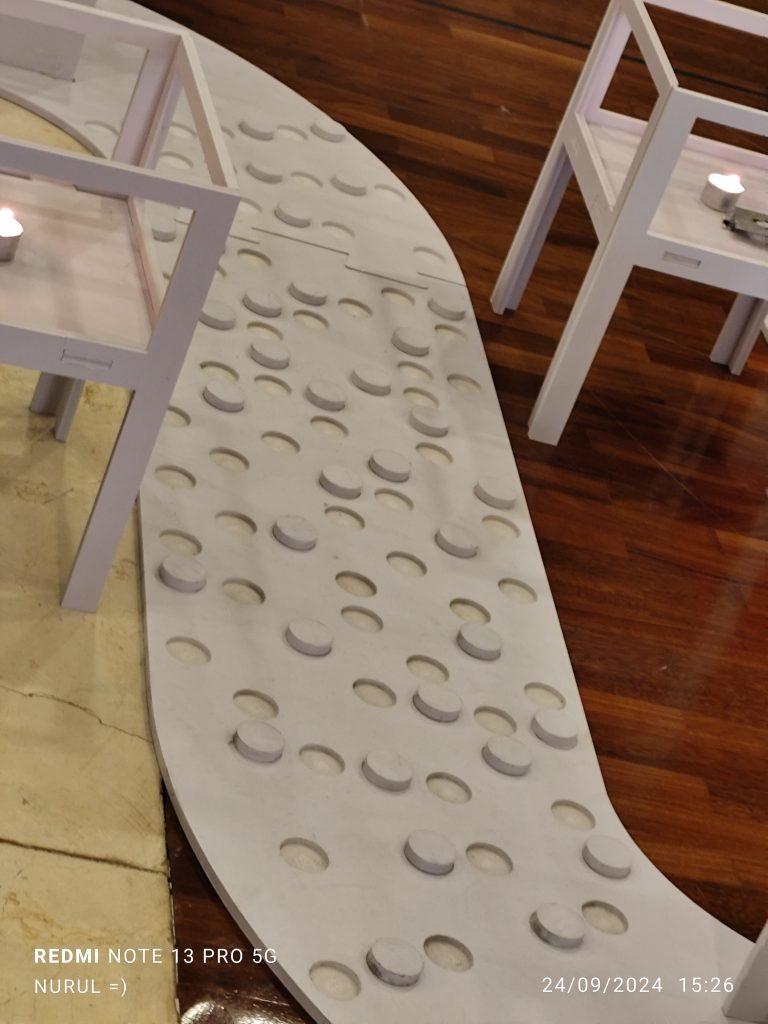
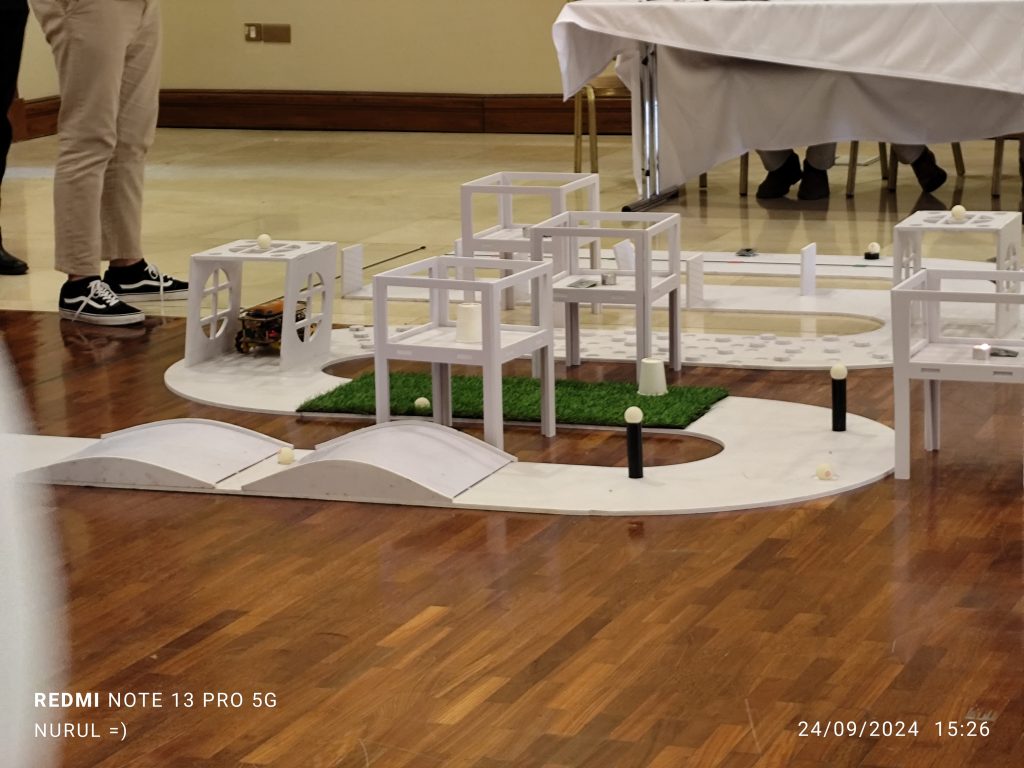
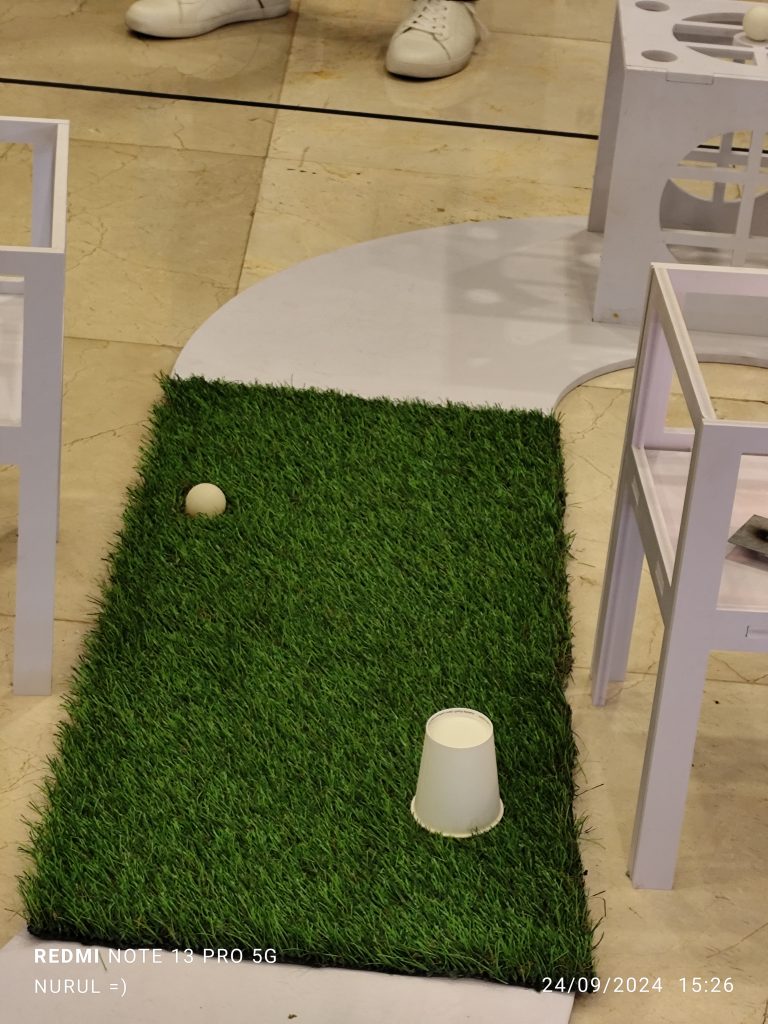
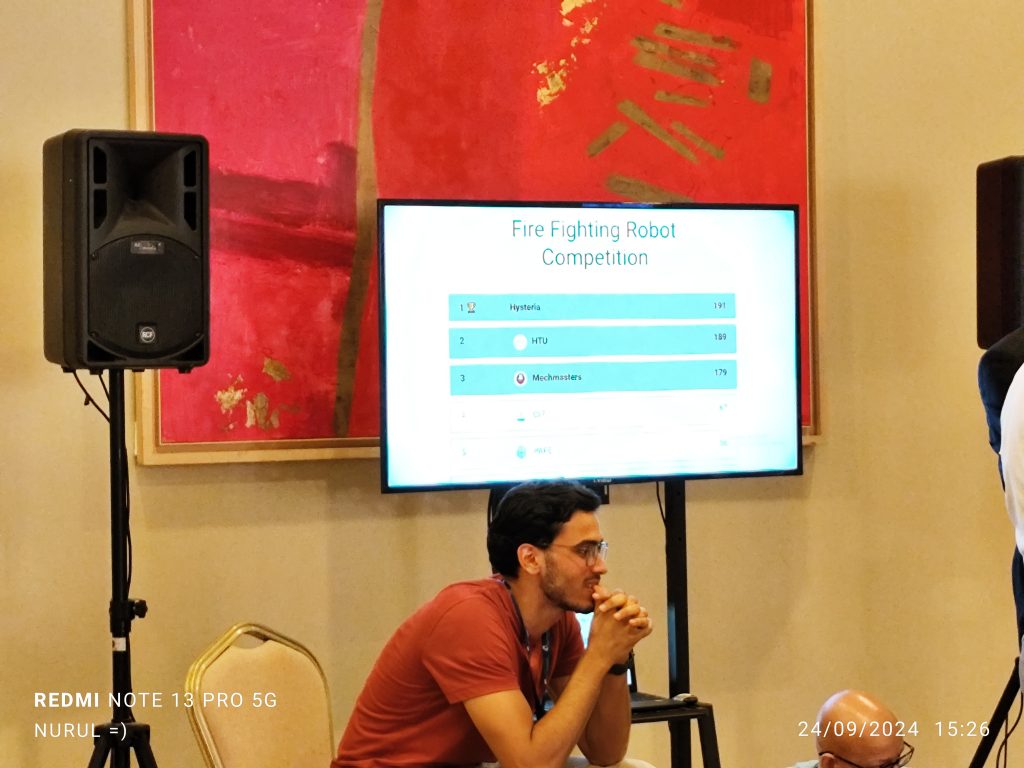
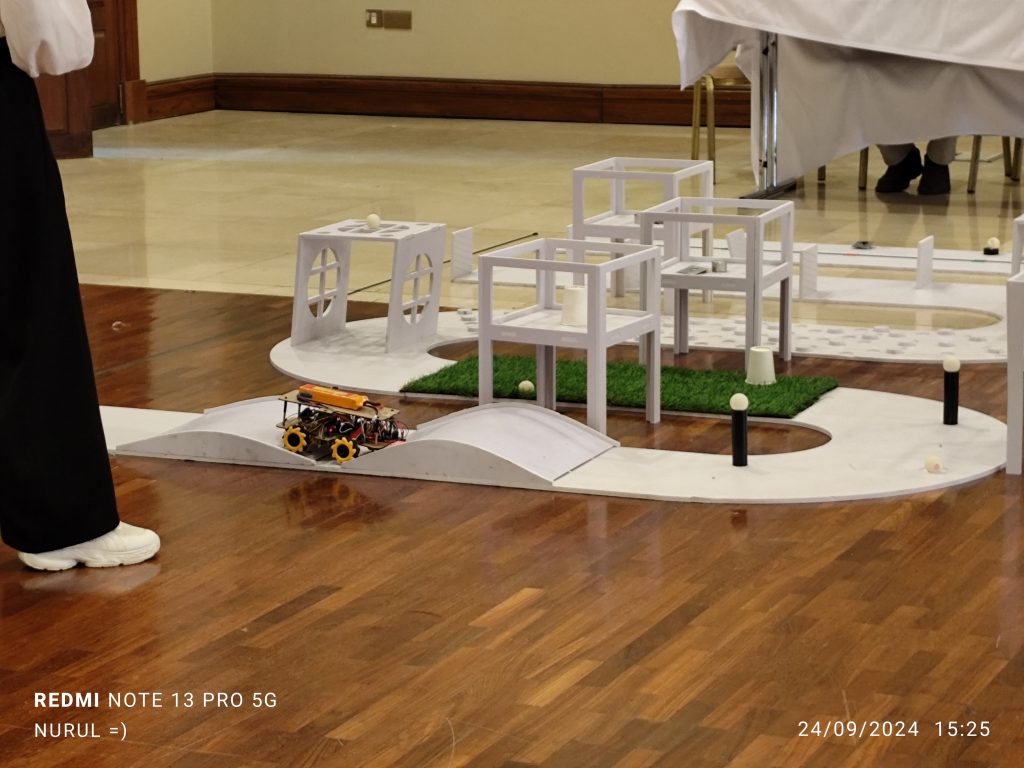
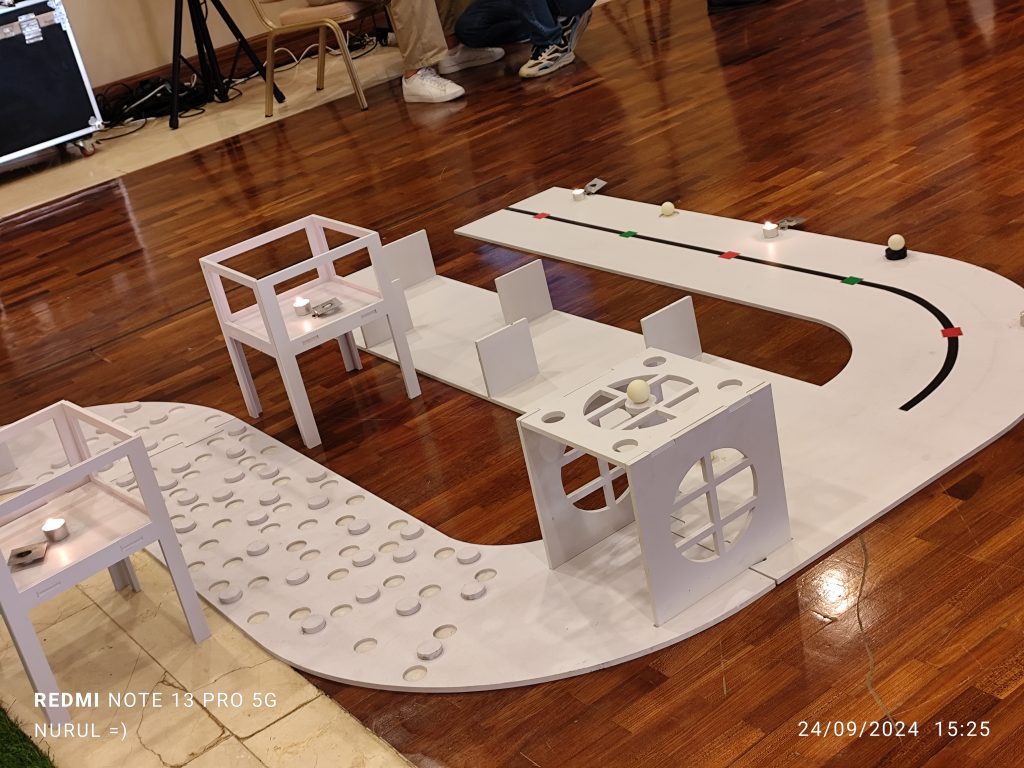
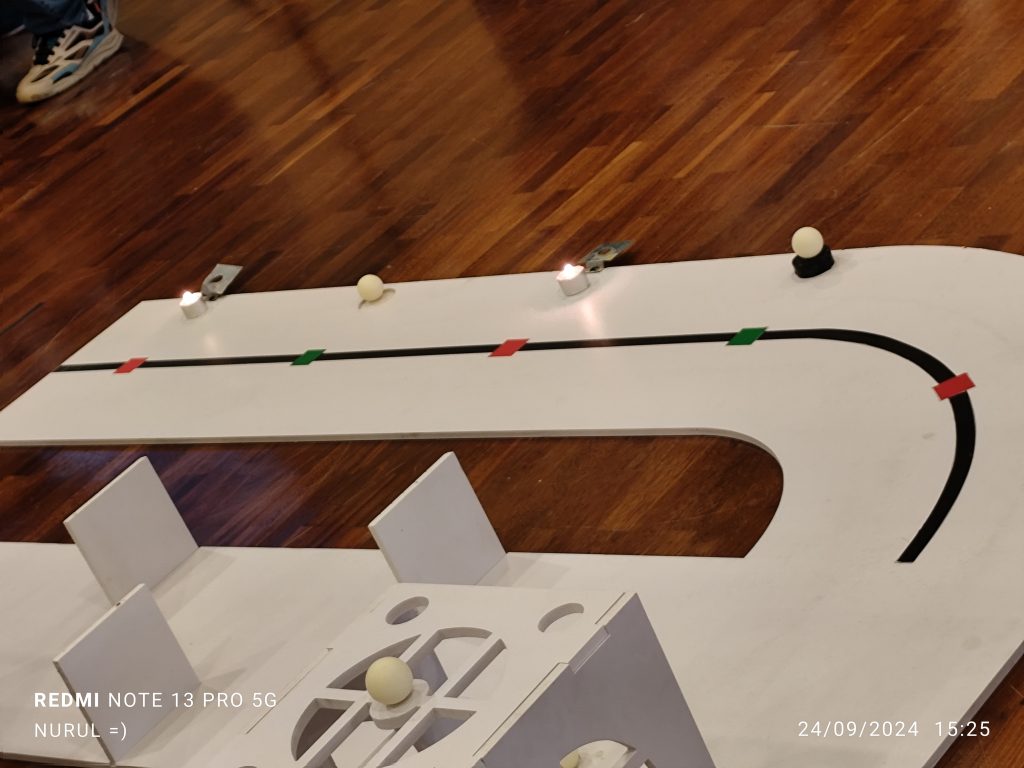
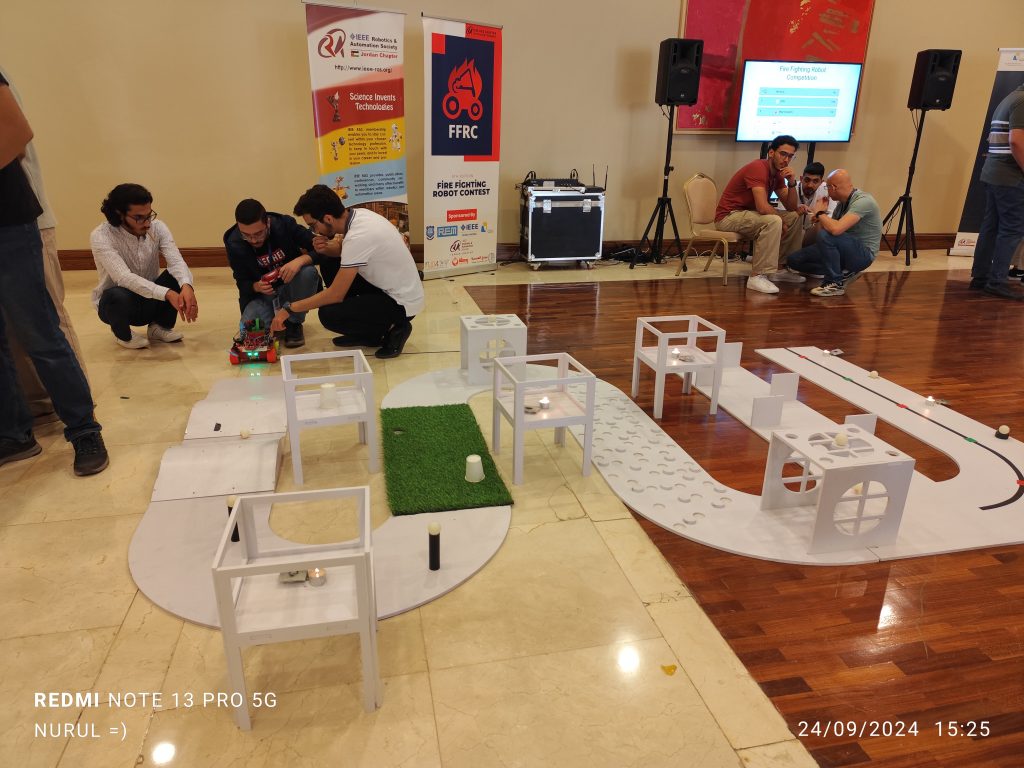
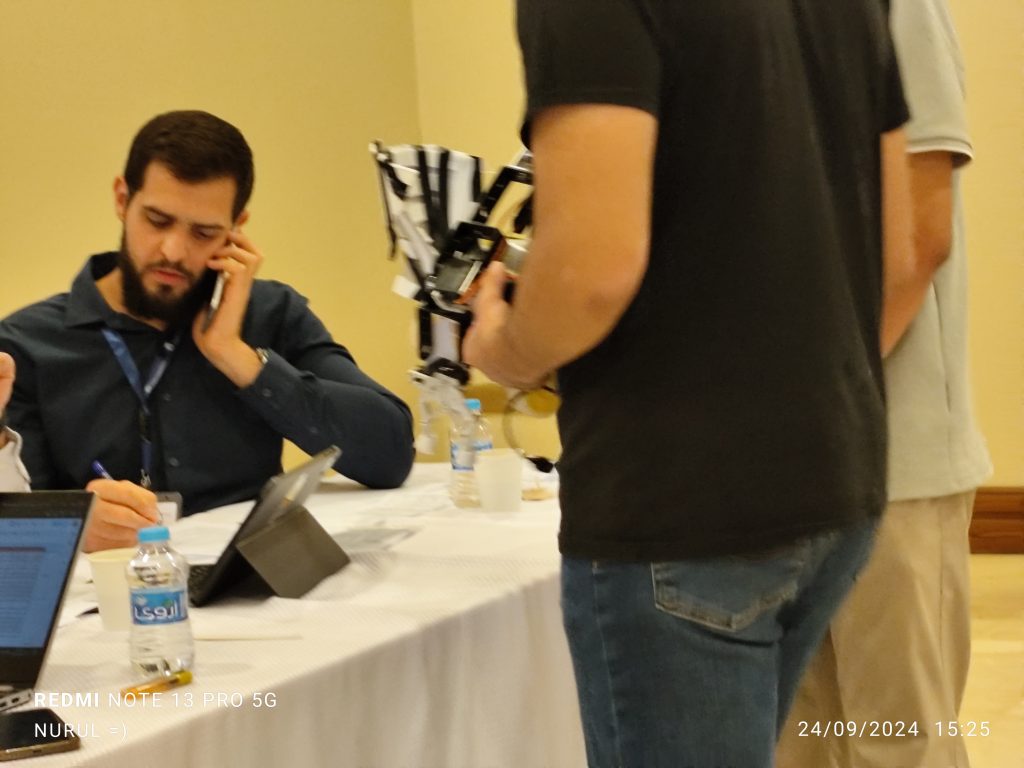
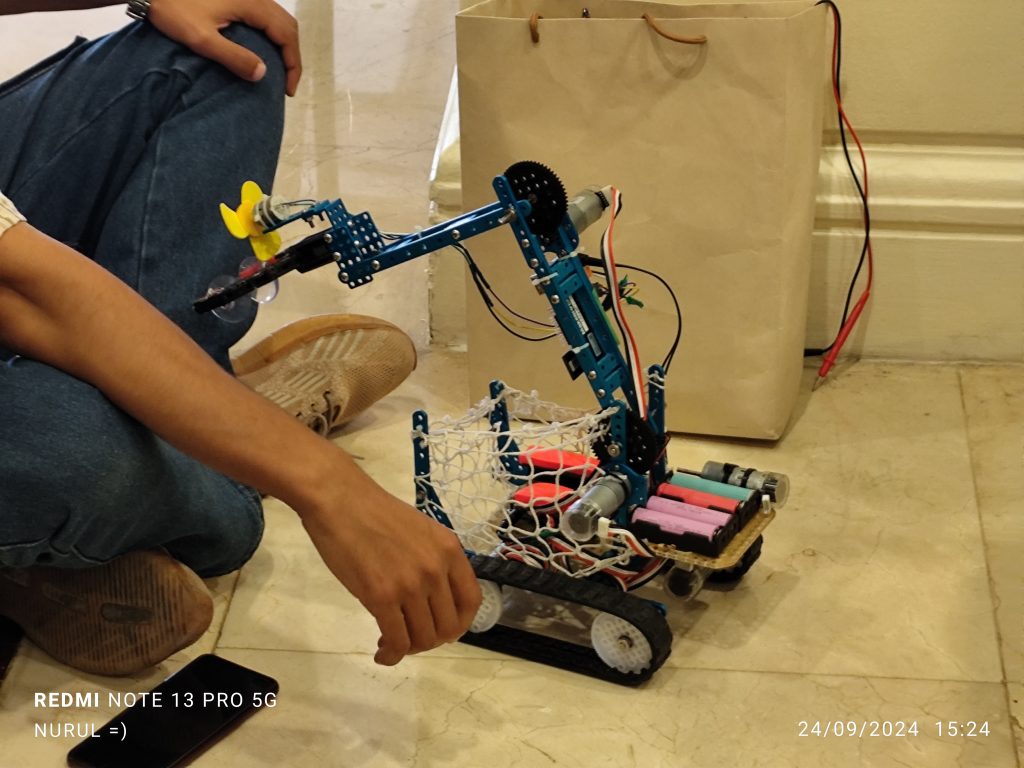
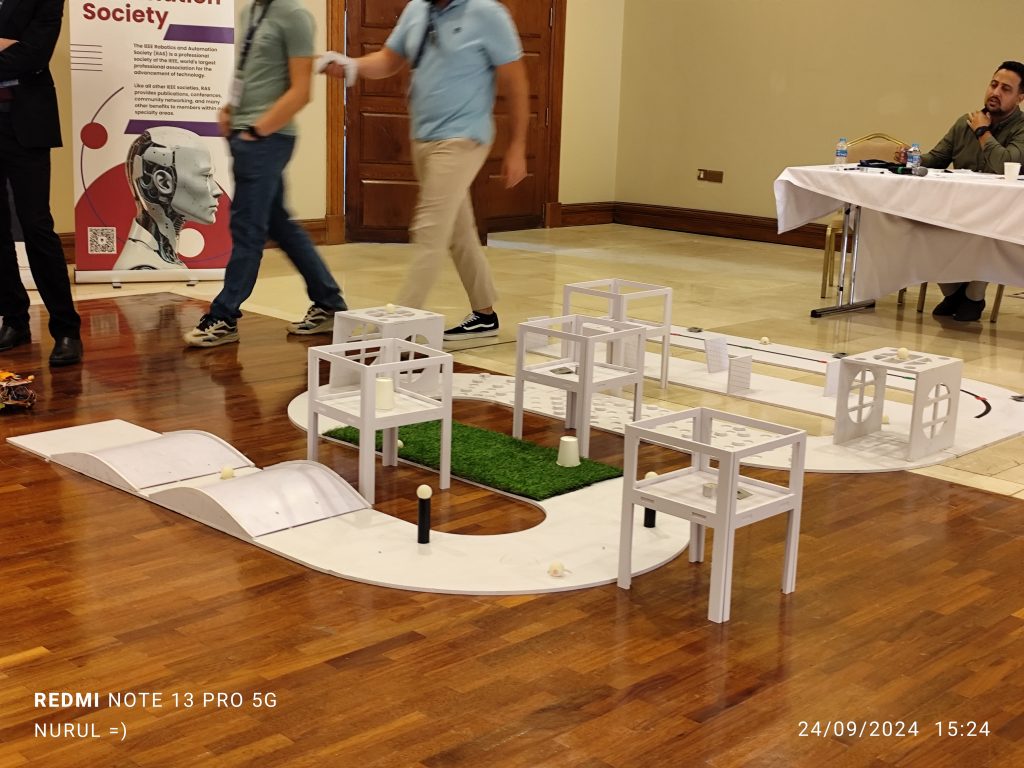
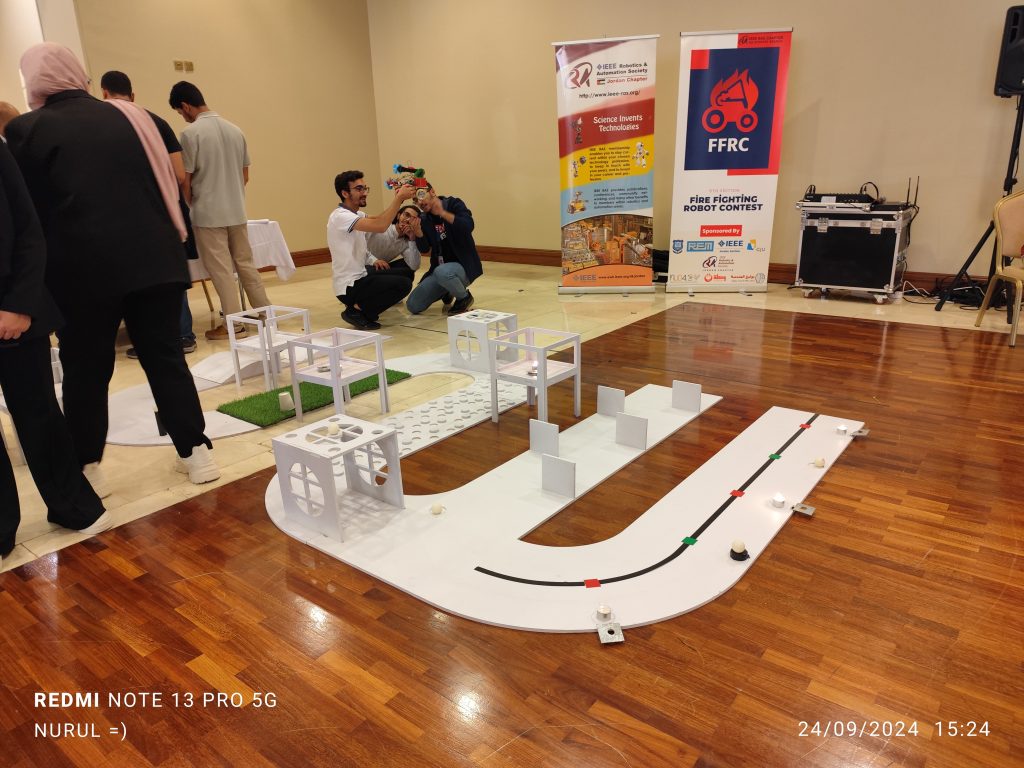