On 17 June 2025, Universiti Malaysia Pahang Al-Sultan Abdullah (UMPSA) organized a prestigious research competition known as CITREX 2025, which brought together local universities from across Malaysia. Regarded as one of the country’s most comprehensive research expositions, CITREX encompasses a wide range of disciplines, showcasing innovation, creativity, and technological advancement in engineering and beyond. The event serves as a valuable platform for the Malaysian research community to connect, while providing an annual opportunity to showcase and evaluate the outcomes of UMPSA researchers’ work, highlighting the university’s research capabilities.
The competition featured various categories, including:
-
Electrical & Electronics Engineering
-
Mechanical Engineering
-
Manufacturing Engineering
-
Engineering Technology
-
Computer Systems & Software Engineering
-
Industrial Science & Technology
-
Industrial Management
-
Chemical & Natural Resources Engineering
-
Civil Engineering & Earth Resources
As a lecturer, I view this research competition as a strategic avenue to amplify the impact and visibility of our faculty’s contributions. It allows researchers to share their findings, explore commercialization potential, and identify income-generating opportunities. CITREX also encourages participants to reflect on two key questions: What is new in this research? And what are the implications for industry and the target market?
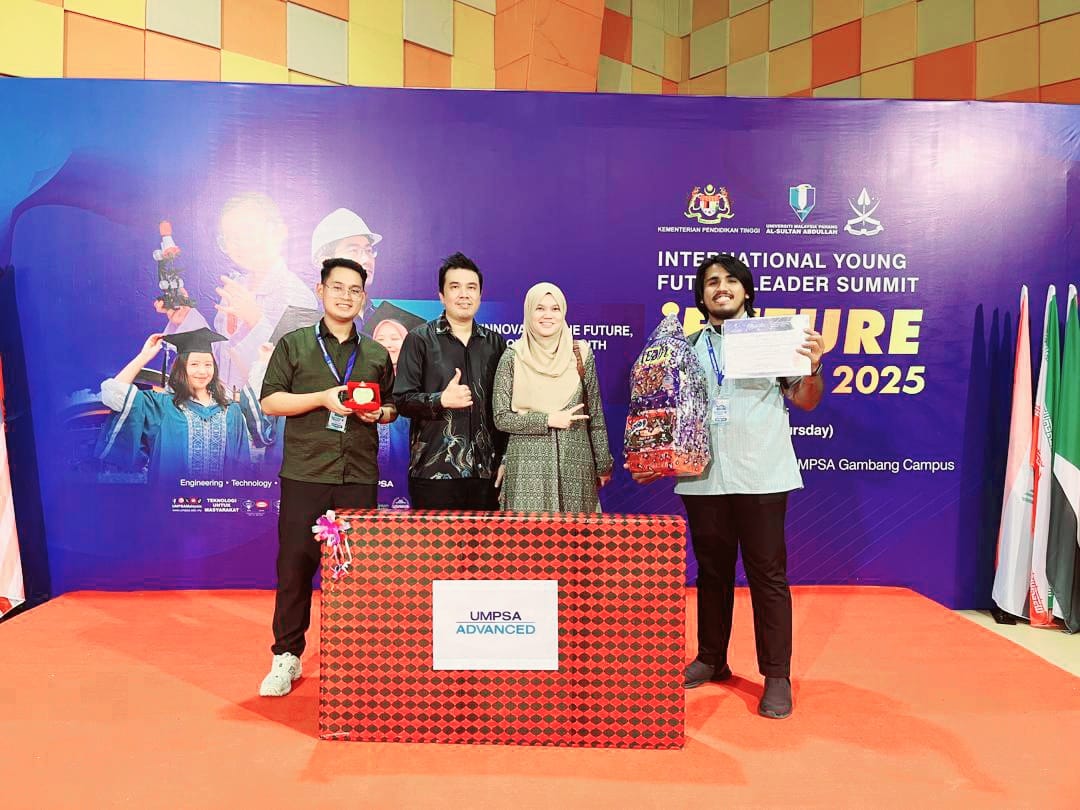
Citrex 2025
I had the opportunity to participate in this year’s competition, and I am proud to share that I was honored with a Gold Award for my product innovation. This recognition not only celebrates the innovation itself but also reinforces the value of contributing research that is both meaningful and impactful to society.
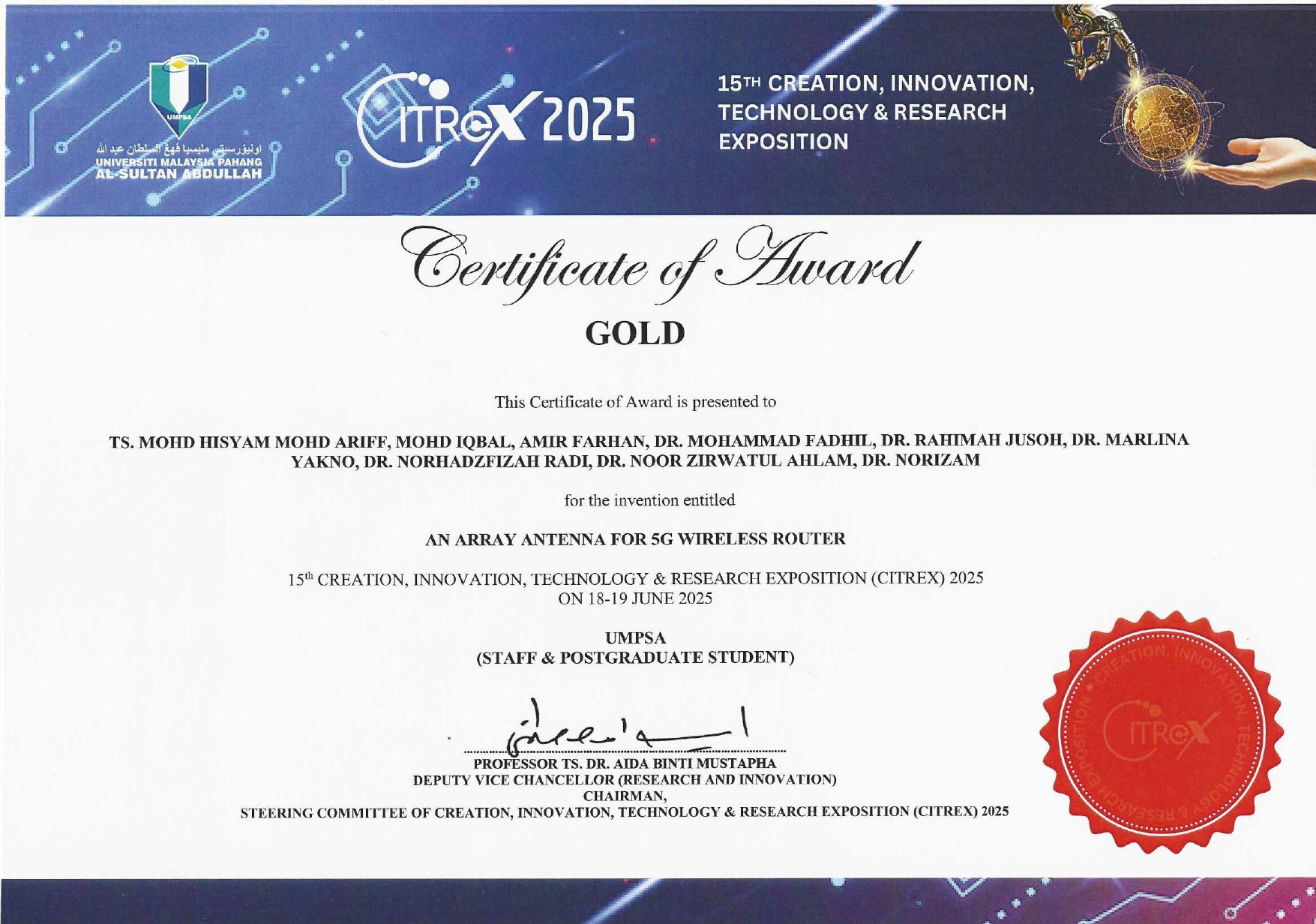
Citrex 2025 Certificate
Leave a Reply